And Yet It Moves! Hygroscopic Movements of Plants
Polish version is here |
The following article was originally published in the journal for educators Biologia w Szkole (eng. Biology in School) (3/2016):
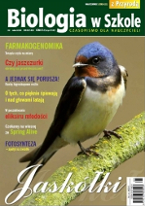
The phrase used in the title of this article (Ital. “eppur si muove”) was allegedly spoken by Galileo—an Italian astronomer, mathematician, physicist, and philosopher who is credited with laying the foundations for modern physics and other exact sciences [1]. According to historical accounts, he uttered these words in 1633 when he was forced to recant his thesis on the Earth's motion around the Sun.
Just as many of Galileo’s contemporaries found it difficult to believe his claims about the movement of the Earth, even today, the fact that plants can move often comes as a surprise—despite being well-documented.
Plants have evolved a variety of movement mechanisms distinct from those used by animals. Animal organisms rely on muscle tissue for movement, which is composed of specialized contractile proteins such as myosin, actin, and others.
Plants can move, but they lack the ability for self-locomotion like animals, meaning they cannot actively relocate their entire body. Unlike animals, plants do not possess muscles, so they have developed alternative movement strategies. These include, among others, growth movements and turgor movements. The former are associated with the expansion of cells forming a given plant organ, while the latter result from changes in turgor pressure, i.e., the tension in the cell wall caused by hydrostatic pressure within the cells.
In most cases, plant movements are too slow or subtle for the naked eye to detect. However, there are many exceptions, such as the folding movements of the sensitive plant Mimosa pudica (Photo 1A) [2] or the trap-leaf movements of carnivorous plants like the Venus flytrap Dionea muscipula and the Cape sundew Drosera capensis (Photo 1B) [3].
In addition to the aforementioned growth and turgor movements, there are also other types of movement. Among the lesser-known yet highly fascinating ones are so-called hygroscopic movements. Conducting observations to confirm their existence is not difficult, even with commonly found plant species.
Observations
Hygroscopic movements can be easily observed in the cones of many coniferous tree and shrub species belonging to the group of gymnosperms Gymnospermae. Pine cones Pinus can be used for this purpose, but due to their availability in my home garden, I conducted my observations on spruce cones Picea.
Female spruce cones are cylindrical and hang downward (Photo 2). Once the seeds mature, the cones fall off intact.
This phenomenon can naturally be observed in the wild, but to examine it under more controlled conditions, a cone should be removed from the tree.
Under moderate air humidity conditions, the cone’s scales are slightly open, as seen in Photo 2.
However, let’s try placing the cone in an environment saturated with water vapor. This can be achieved by sealing the cone in a container with several layers of damp blotting paper at the bottom. Another method is to wrap the cone in a damp paper towel and keep it in a warm place. Within a relatively short time (from a few minutes to several hours), it becomes evident that the previously open scales have now tightly closed together (Photo 3A).
What effect will placing the cone in dry air have? To find out, simply place the cone near a heater or seal it in an airtight container with a desiccant, such as silica gel or a calcium chloride (CaCl2) desiccant. After some time, the cone undergoes a noticeable transformation—the scales stop adhering to one another and spread even wider than before (Photo 3B).
The sequence of images in Photo 4 illustrates how the scales move. In this case, the scales closest to the base of the cone are the first to respond to decreasing humidity.
This process is fully reversible. The scales repeatedly adjust in response to changes in air humidity. In dry air, when the scales are spread apart, simply shaking the cone gently will cause its characteristic seeds to fall out (Photo 5). These seeds have wing-like structures, a clear adaptation for wind dispersal (anemochory).
Another plant species exhibiting hygroscopic movements is the strawflower Xerochrysum bracteatum. This plant is also known as the everlasting flower and belongs to the aster family Asteraceae. Originally from Australia, it is cultivated worldwide as an ornamental plant [4]. Strawflowers are particularly well-suited for dry bouquets, as they retain their natural, fresh colors for an extended period after drying.
The branched and hairy stems of strawflowers typically reach a height of 30–100 cm (12–39 inches). The leaves, up to 10 cm (4 inches) long, are entire and lanceolate.
Like other members of the aster family, strawflower blossoms consist of composite inflorescences known as capitula, which lack a developed calyx and are positioned on enlarged shoot tips (Photo 6). The colorful elements are not petals but bracts that surround the capitulum containing the actual flowers [5].
The mentioned bracts exhibit strong hygroscopic movements: in humid conditions, they close to protect the inflorescence, while in dry conditions, they open. For experiments, it is useful to isolate a single bract (Photo 7).
It is convenient to hold the bract at its base using a small pair of tweezers. The hygroscopic movement of the bract is best observed from the side. In low-humidity conditions, the bract takes on a characteristic curved shape (Photo 8A). When a drop of water is applied at the bend, a movement visible to the naked eye begins (Photo 8B, C). Within a few dozen seconds, the bract assumes the shape seen in Photo 8D.
This process is also reversible—as the bract dries, it returns to its original shape.
Explanation
Growth movements, as they result from uneven cell growth in different regions of an organ, are irreversible. In contrast, turgor movements are usually reversible. However, both types of movement occur only in organs composed of living cells, as these processes require the active participation of metabolically active protoplasts [6].
Hygroscopic movements are not related to changes in turgor pressure or growth. The structures responsible for this type of movement typically consist of dead cells. These cells retain only their cell walls, as they no longer contain protoplasts.
Hygroscopic movements are caused by uneven deformations of the cell wall, which result from changes in the dimensions of the cell walls (shrinking, swelling) due to variations in water saturation. The differential response of cell walls is often linked to differences in the arrangement of cellulose microfibrils within the wall [7].
The mechanism behind hygroscopic movements is similar to the function of certain human-engineered devices, such as bimetallic strips, which deform in response to temperature changes, or bimorph actuators, which bend similarly when subjected to an electric voltage.
Thus, the movement mechanisms observed in the scales of spruce cones Picea and the bracts of strawflower inflorescences Xerochrysum bracteatum are quite similar. Closer observations of strawflower bracts reveal that the bending is not caused by the entire structure but rather by a specific region near its base. This is due to differences in the cellular structure of the upper and lower surfaces of the bract. In fact, this characteristic is common among all plant organs capable of hygroscopic movements [8].
In both described cases, hygroscopic movements serve to protect delicate structures (such as seeds and flowers) from unfavorable environmental conditions. These mechanisms are also frequently used by plants for seed dispersal. For example, this is seen in the opening of soapwort Saponaria seed capsules. The seeds of stork’s-bill plants Erodium—such as the common stork’s-bill Erodium cicutarium, found in Poland—self-burrow into the soil or rather screw themselves into it, thanks to hygroscopic movements [9].
I believe the reader will agree that hygroscopic movements are just as fascinating as other types of plant movement. They play a crucial role in the lives of many plant species and are relatively easy to observe, making them an excellent tool for sparking curiosity about the natural world.
References
- [1] Hall A.R., Galileo nel XVIII secolo, Rivista di filosofia, 15 (1979), pp. 375-83 back
- [2] Ples M., Wstydliwa roślina (eng. Sensitive plant), Biologia w Szkole, 6 (2015), Forum Media Polska Sp. z o.o., pp. 52-56 back
- [3] Ples M., O rosiczce słów kilka, czyli wyhoduj żywą muchołapkę! (eng. A Few Words About Sundews: Grow Your Own Living Flytrap!), Biologia w Szkole, 1 (2016), Forum Media Polska Sp. z o.o., pp. 51-56 back
All photographs and illustrations were created by the author.
Marek Ples